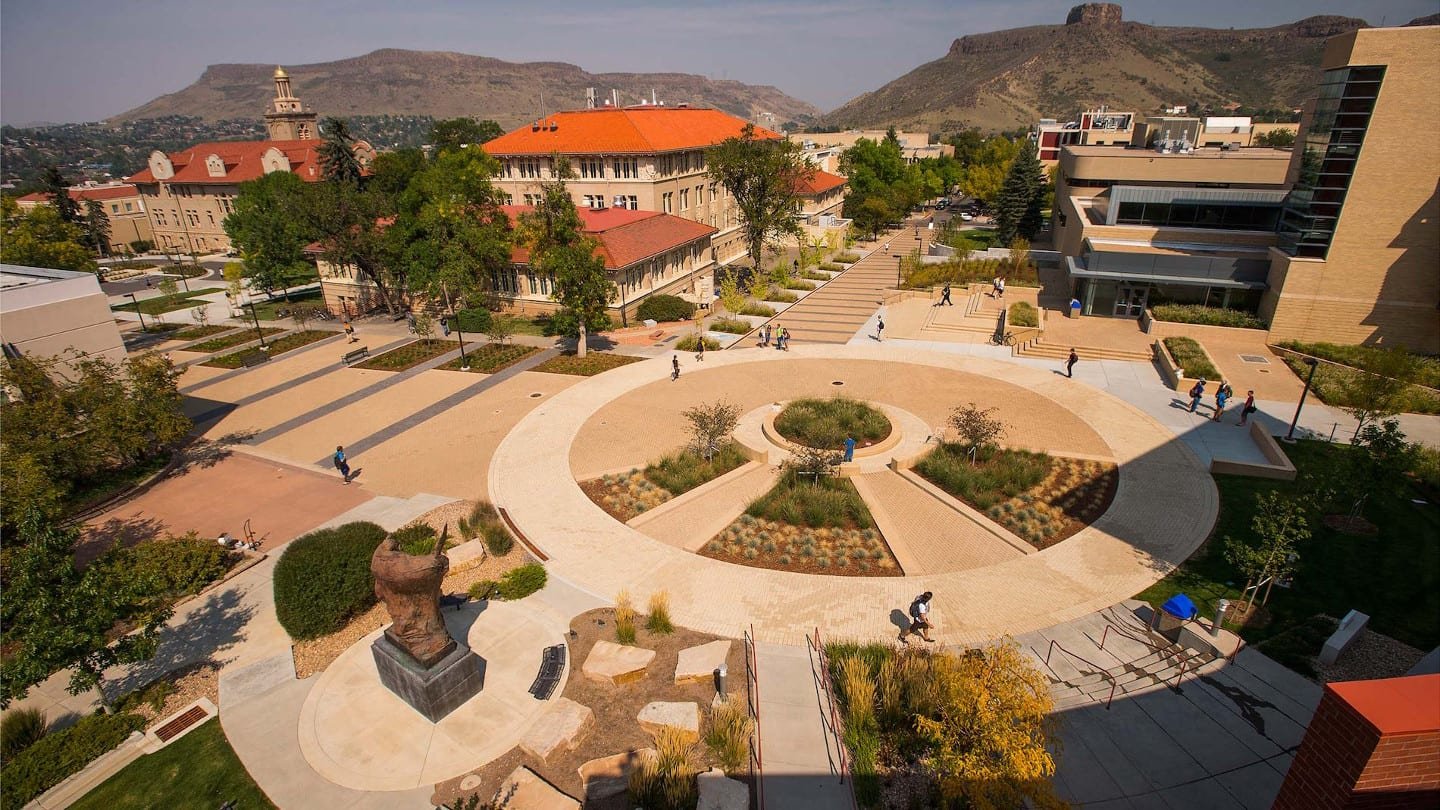
Researching novel solar cell materials
The Context.
When: 10 weeks in the summer of 2015
Where: Colorado School of Mines (CSM)
Context: I spent a summer conducting research through the NSF and CSM-sponsored Renewable Energy Materials Research Science and Engineering Center (REMRSEC) REU program
Team: Under the guidance Dr. P. Craig Taylor (Emeritus Professor, Dept. of Physics) and Lakshmi Krishna (Research assistant professor, physics dept.)
My Role.
I was responsible for analyzing solar cell materials using a technique called electron paramagnetic resonance (EPR) spectroscopy.
In 2015, I was lucky enough to earn a spot in the Colorado School of Mines REMRSEC REU program, which invites STEM undergraduates to conduct research on fundamental material issues related to the use of science and technology in renewable energy.
During my time in Colorado, I worked to characterize several solar cell samples. The experience offered an exciting glimpse into renewable energy research and the intricacies of solar cell efficiency.
Background
During the summer, we researched silicon clathrate samples as potential novel solar cell materials. We also tested a few quantum dot samples using the same method.
Silicon clathrates are cage-like crystalline inclusion compounds, meaning the silicon forms “cages” around compounds called “guests,” in this case, sodium. When sodium is present at high high, the material acts like metal whereas, when sodium is present in low concentration, the material acts a semiconductor. When light hits a semiconductor, the energy from the photons can be absorbed by electrons, allowing light to be converted into an electrical current. This is one reason why silicon-sodium clathrates with low enough levels of sodium doping to be semiconductors are of interest for advanced solar cell materials.
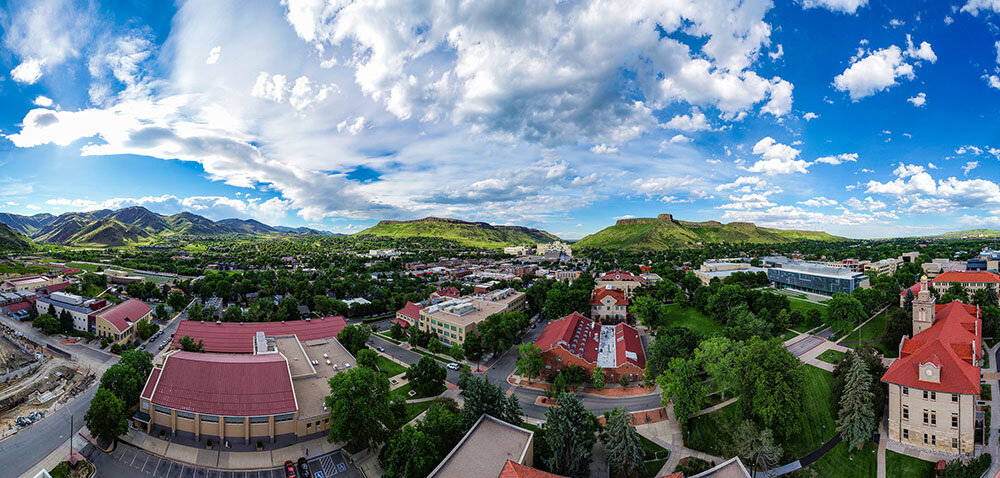
Silicon-clathrate solar cells can increase solar panel efficiency by re-capturing lost wavelengths of light.
Electrons orbiting an atom. Electrons closest to the atom core (nucleus) are in lower energy levels than those in the outer-ring
Most solar cells possess smaller bandgaps, allowing them to absorb wide ranges of the solar radiation that hits Earth. However, they miss large swaths of the higher-energy light spectrum. Panels comprised of materials with varying bandgaps are able to absorb wider ranges of electromagnetic radiation, thus improving solar panel efficiency. Silicon-sodium clathrates have wide bandgaps, and so their use in solar cells is aimed at re-capturing typically lost wavelengths of light.
Electrons orbit nuclei in energy levels called shells. The outer most shell is known as the valence shell, which comprises a band of energy levels. This is why this shell sometimes also called the valence band. If an electron absorbs enough energy, say from light shining on it, it can escape the valence band and move to the conduction band. In this state, the electron is able to move freely throughout the material. The energy required to make this jump is called the bandgap. In materials with small bandgaps, electrons having an easier time making this transition, thus making that material more conductive. Conversely, materials with high bandgaps are strong insulators. Since a light ray’s energy is inversely proportional to its wavelength, a compound’s bandgap determines which wavelengths of light it can absorb.
Diagram of a bandgap
I was responsible for analyzing the properties of the silicon clathrate samples synthesized by the Carnegie Institute of Washington using high pressure techniques. Our main goal was to develop a method to grow silicon clathrates with just the right level of Na doping to be used as a solar cell.
High and low energy states of electrons in increasing magnetic field
EPR methodology applies a constant electromagnetic field with an increasing magnetic one (right). As the magnetic field intensifies, the energy difference between the high and low states does as well. When this energy difference matches the energy of the incoming electromagnetic radiation, resonance occurs, causing a peak in the absorption spectrum. By reading the sample’s absorption spectrum, we can determine the sodium levels present.
For this analysis, I used a technique called electron paramagnetic resonance (EPR), which allows researchers to probe the microstructure of samples with unpaired electrons, like sodium. Electrons have a property called a magnetic moment, and when placed into an external magnetic field, their magnetic moment either aligns with or against the field, thus creating a low or high energy state (left).
EPR Apparatus Diagram
End Results
At the end of summer, our preliminary results indicated that the samples were still over-doped. Although we didn’t find that sodium sweet-spot in the short timeframe we had, it was an exciting dive into the world of renewable energy, and it taught me important lessons about persistence, optimism, and finding purpose, even in difficult situations.
Example of data taken in the lab. Our sample (blue) had two peaks, indicating the clathrate was doubly-occupied. (Standard sample with single occupancy shown in red)
Structures of silicon clathrates with single (left) and double (right) sodium occupancy
Why it matters.
Climate change poses a serious threat to human safety, prosperity, and international peace. Rising seas, harsher storms, struggling agricultural yields, scorching heat waves and drought represent only a few risks posed by carbon emissions. By improving the efficiency of solar cells, we can help cut emissions from natural gas and oil.
On a personal note, improved solar means better options for people in my home community, the Bay Area, to get off-grid, an increasingly sought-after option amid brutal fire seasons and frequent, rolling blackouts.
Want to learn a little more physics? You can read more about my work in the research poster below, which I presented at the REMRSEC student research symposium at the end of the summer.